
There are different types of batteries but Lithium-ion batteries are the most popular batteries playing an important role in present battery revolution. Lithium-ion batteries are successful because Lithium is an ideal material for energy storage applications because it has the greatest electrochemical potential and provides the largest energy density for its weight [2].
Lithium-ion batteries have many advantages over other types of batteries existing in the market.
Key advantages of Lithium ion battery over other battery technologies
Long lifetime
Experiencing very low self-discharge rates
Large choice of cell designs and battery chemistries
Highest energy densities among all the state-of-the-art battery storage technologies.
Better cycling performances, typically thousands of charging/discharging cycles.
Highly scalable and it can be adapted to practically any voltage, power and energy requirement
Main components of a Li-ion battery:
Li-ion battery technology requires four main components: cathode, anode, electrolyte, and separator to function properly.
In Li-ion battery, cathode is usually made up of lithiated metal oxide (LiCoO2, LiMO2, etc.), while the anode is mostly made of carbon material, and electrolyte is made up of lithium salts (such as LiPF6) dissolved in organic carbonates.
How does Lithium-ion battery work?
During the charging cycle of the battery, the lithium atoms in the cathode become lithium ions and migrate through the electrolyte toward the carbon anode where they are deposited between carbon layers. This process is reversed during discharge. Because lithium reacts to water, non-aqueous solutions are used.
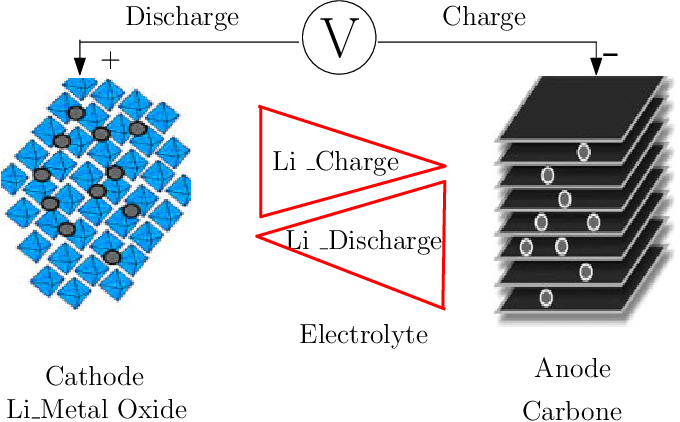
Lithium-ion is not a single battery type
It’s important to understand one thing about Li-ion batteries that Li-ion is not a single battery type rather Li-ion covers the broad range of technology depending on what chemistries battery got on the cathode side and anode sides. Mostly cathodes have different chemistry variations and they are usually paired with carbon based anodes.
Also different chemistries on the electrodes can have completely different energy densities, power densities, cycle life, calender life, and cost e.t.c.
Main chemistry variations falling under the family of Li-ion batteries:
Lithium Cobalt Oxide(LiCoO2) — LCO
Lithium Manganese Oxide (LiMn2O4) — LMO
Lithium Iron Phosphate(LiFePO4) — LFP
Lithium Nickel Manganese Cobalt Oxide (LiNiMnCoO2) — NMC
Lithium Nickel Cobalt Aluminum Oxide (LiNiCoAlO2) — NCA
Lithium Titanate (Li2TiO3) — LTO
Lithium Ferro Phosphate (LFP)
· Energy density: (★★) 2/5
· Power density: (★★★★) 4/5
· Cycle life: (★★★★) 4/5
· Safety: (★★★★★) 5/5
· Cost: (★★★★) 4/5
Lithium Ferro Manganese Phosphate (LFMP)
· Energy density: (★★★) 3/5
· Power density: (★★★★) 4/5
· Cycle life: (★★★★) 4/5
· Safety: (★★★★★) 5/5
· Cost: (★★★★★) 5/5
Lithium Nickel Cobalt Manganese (NCM 333 or 111)
· Energy density: (★★★) 3/5
· Power density: (★★★) 3/5
· Cycle life: (★★★★) 4/5
· Safety: (★★★★) 4/5
· Cost: (★★) 2/5
Lithium Nickel Cobalt Manganese (NCM 523)
· Energy density: (★★★★) 4/5
· Power density: (★★★) 3/5
· Cycle life: (★★★) 3/5
· Safety: (★★★) 3/5
· Cost: (★★★) 3/5
Lithium Nickel Cobalt Manganese (NCM 622)
· Energy density: (★★★★) 4/5
· Power density: (★★★) 3/5
· Cycle life: (★★★) 3/5
· Safety: (★★★) 3/5
· Cost: (★★★) 3/5
Lithium Nickel Cobalt Manganese (NCM 712)
· Energy density: (★★★★★) 5/5
· Power density: (★★) 2/5
· Cycle life: (★★) 2/5
· Safety: (★★) 2/5
· Cost: (★★★★) 4/5
Lithium Nickel Cobalt Manganese (NCM 811)
· Energy density: (★★★★★) 5/5
· Power density: (★★) 2/5
· Cycle life: (★★) 2/5
· Safety: (★★) 2/5
· Cost: (★★★★) 4/5
Lithium Nickel Cobalt Aluminium (NCA)
· Energy density: (★★★★★) 5/5
· Power density: (★★★) 3/5
· Cycle life: (★★★) 3/5
· Safety: (★★) 2/5
· Cost: (★★★★) 4/5
Traditionally focus has been on improving the cathodes. They are usually paired with graphite anodes, but more recently a mixture of graphite with silicon has been used to achieve higher energy density. However, the use of silicon comes at the cost of reducing cycle life, due to swelling of the anode.
How can energy density of Li-ion battery be improved?
In Li-ion batteries, lithium ions are stored in active materials acting as stable host structures (known as cathode and anode) during charge and discharge. Therefore, finding host structures (known as cathode and anode) that can accommodate huge number of lithium atoms in them would significantly increase the energy density of the battery.
For example, replacing graphite anodes with silicon and lithium metal because of their extremely high theoretical specific capacities (such as 4000 mAh g−1 for silicon, and 3860 mA h g−1 for Lithium (Li) metal) compared to that of 372 mAh g−1 of graphite could make a huge difference in the energy density of the battery. But replacing graphite with silicon doesn’t come without serious challenges, even though silicon does way better job at absorbing lithium ions than graphite but it goes through severe volume expansion which keeps away silicon from being commercially adopted as an alternate to graphite.
Tesla is making low cost a major priority and is going to use pure low-cost silicon encapsulated with elastomeric polymer to contain the silicon as it breaks apart due to expanding and then contracting as lithium ions move in & out. The optimum concentration of encapsulated silicon particles is 20 – 30%. The size range of the graphite particles is 10 to 40 micron and an average of 8 micron for silicon particles. Graphite will be used in anodes of mass-produced batteries for years to come. Pure silicon volume expansion when loaded with lithium is 300% or 50% if expressed as particle diameter.
There are several techniques to make hybrid graphite / silicon nanoparticles etc., but these techniques are too expensive for Tesla’s current business plans even though better performance is possible. Several different options for increasing the energy capacity of the anode using special types of silicon but the cost of the anode is higher.
How can cycle life of Li-ion battery be improved?
In Li-ion batteries, lithium ions are stored in active materials acting as stable host structures (known as cathode and anode) during charge and discharge. Every time lithium ions are deposited between those host structures (known as cathode and anode) during charging/discharging cycles, those host structures go through severe mechanical degradation limiting the cycle life of Li-ion battery. Therefore, finding host structures (known as cathode and anode) that can accommodate lithium atoms in them and their structure could be able to allow lithium ions to go in and out of the material without compromising the structural integrity of the material would significantly increase the cycle life of the battery.
For example, most families of lithium-ion battery technology use layered transition metal electrodes, most commercial being NMC 811 (each digit represents the ratio of nickel, manganese, and cobalt, respectively, in the mix) which goes through severe mechanical degradation during charging/discharging cycles. One possibility of improving the cycle life of the lithium-ion battery is by substituting layered electrodes with something structurally stronger. For instance, the 100-year-old Swiss battery company Leclanché is developing a technology that uses lithium iron phosphate (LFP), which has an “olivine” structure, as the cathode, and lithium titanate oxide (LTO), which has a “spinel” structure, as the anode. These structures are better at handling the flow of lithium ions in and out of the material [1].
New Developments in Electrolyte
The other important thing is electrolyte which acts as a pathway for lithium ions between the electrodes. Unfortunately the liquid compounds which are good at transporting lithium ions can catch fire at low temperatures. One solution is to use solid electrolytes. But that means other compromises. It’s much harder to transfer anything from solids. Safer polymer electrolytes could be an alternative to the high flammable organic liquid electrolytes.
What does the future hold for Lithium-ion battery technology?
Amidst the high expectations, battery storage is still not ready for the prime time and there is a need for a structural battery research renaissance to further lower the cost of technology, increase the energy density and improve the lifetime of battery technology.
To put it more concretely, there is a need for development and application of advanced characterization techniques such as In situ transmission electron microscopy to better understand the battery process and design better battery system (i.e. optimizing battery materials, understanding cell degradation mechanisms, and ultimately improving the overall battery performance).
Nevertheless, Lithium-ion batteries are anticipated to have a high rate of deployment in the coming decades. Ideal applications of Lithium-ion batteries would be energy storage systems for renewables and transportation.
The downside of Lithium-ion batteries is that Lithium-ion batteries have significant associated adverse impacts, including human rights and pollution impacts during mining, fire risk, and are a future waste management challenge owing to the lack of established recycling systems. Therefore, planning and decision-making influencing the deployment of current and future Lithium-ion battery technologies need to acknowledge and manage these short and longer-term impacts as they pose a significant risk to the viability of the industry and could hinder the transition to a renewable energy system.
References:
https://qz.com/1588236/how-we-get-to-the-next-big-battery-breakthrough/
https://www.advancedsciencenews.com/what-comes-after-the-lithium-ion-battery/
Comments