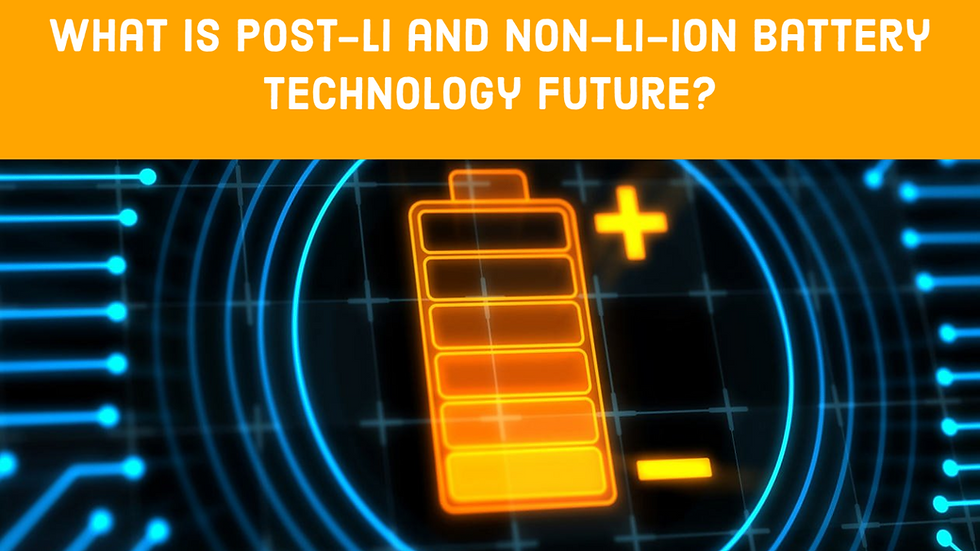
Firstly, It is clear that despite Li-ion batteries have improved the state-of-the-art battery storage technology and has many advantages over other battery technologies today but still Li-ion is not a clear winner in the race to power mobility and utility scale stationary storage applications. Therefore, there is a need to further improve Li-ion batteries and also develop other high performance batteries.
Secondly, need for a variety of fit-to-purpose batteries to satisfy the requirements of broad range of applications, and availability and price issues of Li-ion basic raw materials have stimulated the research interest and development of other types of batteries. Some of the promising post-Li and non-Li-ion battery technologies include:
Solid-state batteries with Li metal anodes.
Lithium-sulfur batteries (Li-S).
Sodium-ion batteries (Na-ion).
RedOx flow batteries, including these with organic shuttles (organic RedOx).
Multivalent ion batteries, based on e.g. Mg2+, Zn2+, Ca2+ and Al3+, f).
Metal-air batteries, including Li-air, Na-air, Mg-air, Al-air, Si-air, Fe-air and Zn-air.
Solid State Battery Technology
The key difference between Li-ion batteries and solid-state batteries is that solid-state batteries replace organic liquid electrolytes of Li-ion batteries with Li-ion conducting solid electrolyte. On the electrodes side, solid state batteries can have conventional intercalation electrode materials, currently used in the conventional Li-ion batteries or can have completely new materials like thin layer of metal lithium serving as an anode.
Why Solid state batteries?
Improved safety (no flammable, toxic or corrosive electrolyte present).
Possibility to have higher gravimetric and volumetric energy densities, i.e. up to 700 Wh/kg and 1400 Wh/l (thanks to high specific capacity of Li metal anode).
Ability to have a wider operating cell voltage window because many solid electrolytes are chemically more inert and more stable over a larger potential window, enabling also the use of high-voltage cathode materials).
Higher power density (related to wider operating cell voltage window as well as to higher thermal conductivity) enabling fast charging.
Broader operational temperature range, also due the ability to operate at elevated temperatures up to >100°C.
Longer shelf life (low self-discharge rate)
Difficulties and Challenges of solid state batteries
Finding a solid electrolyte that can exhibit sufficient conductivity for Li+ (at least >10-4 S/cm and better >10-3 S/cm), while being non-conductive electronically <10-12 S/cm (i.e. Li transference number as close as possible to 1).
Finding a solid electrolyte that must be chemically and electrochemically stable in contact with Li metal and cathode materials in a broad range of operating temperatures and cell voltages.
From the point of view of manufacturing, handling of metallic lithium poses great concerns.
On ground applications of solid state batteries
Bolloré is the only company who has introduced solid state batteries with lithium metal anode to the mobility market and stationary energy storage applications. Bolloré Li Metal Polymer (LMP) batteries use polymer based solid electrolyte, LiFePO4 (LFP) cathode. The use of LiFePO4 (LFP) cathode operating at a low voltage of 3.6 V explains why LMP battery has a relatively low energy density of 120 Wh/kg at the pack level and 240 Wh/kg at the cell. LMP battery also require higher temperatures i.e. 60-80°C to operate because of the limited ionic conductivity of polymer based solid electrolyte at room temperature. Therefore there is a need to find ways to incorporate high-capacity electrode materials with well-functioning solid state electrolytes to unlock full potential of solid state batteries.
How close are solid state batteries?
Solid state batteries definitely promise step change for batteries in terms of safety, improved energy densities, enabling faster charging rates. But in reality solid state batteries are not yet ready for the prime time because they have many engineering and manufacturing challenges that need to overcome before wide commercialisation of these batteries is possible. It is commonly agreed that a noticeable market uptake of the solid state batteries with lithium metal cannot be expected before 2030, especially in electrification of mobility and stationary energy.
Lithium-Sulfur Battery Technology (Li-S)
Lithium-sulfur (Li-S) battery technology is another interesting and promising battery technology. Lithium-sulfur (Li-S) battery technology use sulfur in the positive electrode and metallic lithium as the negative electrode.
The working principle of Lithium-sulfur (Li-S) battery technology is very complicated and somehow still under debate. Because unlike the conventional intercalation type electrode materials used in traditional Li-ion batteries, Lithium-sulfur (Li-S) battery technology use active materials that works via alloying and conversion reactions. This is complicated because unlike the materials intercalation reactions occurring with minor modifications of the crystal structure, the alloying and conversion reactions will completely reconstruct the crystal structure of the host material to form new phases.
In simple words, unlike Li-ion batteries, which need host structures for storing lithium ions during charge and discharge. Lithium-sulfur (Li-S) batteries need no host structures. While discharging, the lithium anode is consumed and sulfur transformed into a variety of chemical compounds; during charging, the reverse process takes place.
Why Lithium-sulfur (Li-S) battery technology?
Low cost is the biggest advantage of Lithium-sulfur (Li-S) battery chemistry attributed to the price of sulfur and world wide availability particularly when comparing to other cathode materials.
Li-S batteries can have a high gravimetric capacity (theoretical capacity of the sulfur cathode is 1,675 mAh/g).
Environmental battery friendly compared to lithium-ion batteries as they do not require heavy metals such as cobalt and are free of critical raw materials.
Difficulties and Challenges of Lithium-sulfur (Li-S) battery technology
Electrolytes are the central problem of Li-S batteries. As conventional electrolytes cannot be used, there is a need to find electrolytes that can fulfil the requirements for Li-S batteries.
From the point of view of manufacturing, handling of metallic lithium poses great concerns.
Sulfur as cathode material is non-electrically conductive, so it requires significant amounts of additives (typically carbon materials) up to 20-30wt.%, which reduces the amount of active material in the electrode.
Big gaps in research and development to be addressed by academic researchers before advancing to industrial scale production.
Insights into phase transformation, mechanical degradation, and SEI layer evolution are required for exploiting the full potential of Lithium-sulfur (Li-S) battery technology.
How close are Lithium-sulfur (Li-S) battery technology?
Saft, a French battery manufacturer, claims that major technical challenges for Lithium-sulfur (Li-S) battery technology have already been overcome and Lithium-sulfur (Li-S) battery technology quickly heading towards full scale prototypes. But if we are talking about applications requiring long battery life, we are at least 5 years away from making it happen.
Sodium-ion Battery Technology (Na-ion)
The sodium-ion battery works exactly similar to that of Li-ion batteries. The key difference between sodium-ion battery and Li-ion batteries is that In sodium-ion battery, instead of lithium ions, sodium ions (Na+) ions are stored in active materials acting as stable host structures (known as cathode and anode) during charge and discharge.
The cell design of Na-ion batteries is also similar to that of Li-ion – in short, the cell is composed of a cathode containing a sodium compound and an anode able to accept the sodium atom. Despite many chemical similarities of Li+ and Na+ ions, the latter one has a larger ion radius, causing that graphite, the dominant active anode material in Li-ion batteries cannot be used in Na-ion chemistry due to a very low Na+ ion storage capacity.
Why Sodium-ion battery Technology?
Sodium is a cheaper alternative to lithium and the sixth most abundant element on the planet (security of future supplies).
Sodium-ion batteries can significantly lower battery technology cost.
Generally, Na-ion batteries are considered environmentally friendly because they do not contain elements like Co, Ni, Mn, Cu, and Li.
Difficulties and Challenges of Sodium-ion battery Technology
Development of suitable active materials (i.e. electrodes, electrolytes)
How close are Sodium-ion battery Technology?
The technology readiness level of Na-ion batteries depends on the development of active materials, cell designs and validation of manufacturing processes. It is still in the learning phase. Commercialising sodium-ion batteries is expected to begin in the next five to 10 years.
RedOx flow Battery Technology
Redox flow batteries consist of two tanks of liquid commonly called electrolytes or working fluids. When pumped into a chemical reactor, chemical energy of working fluids is converted into electrical energy through reversible oxidation and reductions reactions back and forth during charging and discharging.
Redox Flow batteries offer a variety of benefits. The most singular advantage redox flow batteries have other battery technology is the architecture of redox flow batteries that allow decoupling of energy rating and power rating which means that power and energy capacity of the system can be scaled independently from each other by separate sizing of the tank volume and the cell stacks (reaction cells).
Why redox flow batteries?
Decoupling of energy rating and power rating i.e. the power and energy capacity of the system can be scaled independently from each other by separate sizing of the tank volume and the cell stacks (reaction cells).
Common flow batteries rely on aqueous electrolytes that are not flammable, and therefore a safe battery operation is guaranteed.
Discharge 100% of the stored energy each cycle without losing any capacity.
Do not degrade for more than 20 years.
Difficulties and Challenges of redox flow batteries
Vanadium redox flow battery, most advanced and close to commercialization amongst redox flow battery chemistries, suffers from high cost of the vanadium and relatively low energy densities (i.e. 25 Wh/L).
How close are redox flow batteries?
Redox flow batteries are considered to be the promising candidate, particularly for the grid scale battery storage applications due to its exceptional scalability and flexibility to improve the stability, efficiency, and sustainability of the power grid.
Most commonly used chemistries for redox flow batteries are based on materials like vanadium, zinc, and iron. Vanadium and Zinc-based flow batteries are the most advanced and close to commercialization amongst redox flow battery chemistries, but high cost of the vanadium and relatively low energy densities (i.e. 25 Wh/L) are still some key challenges standing in the way of further commercial and industrial application of Vanadium RedOx Battery (VRB).
Commercialization of a battery technology takes time
It is good to see that there is a lot of interest in the research and development of future battery technologies. But the problem is that the commercialization of a battery storage technology is a slow process. For instance, it took 40 years to get the current lithium-ion batteries to the current state of technology. Most of these post-Li and non-Li-ion battery technologies are still in the lab scale and would take a lot of time before could compete with current lithium-ion batteries. It is clearly known in the battery circles that once you have a breakthrough in any battery technology, you need probably three years to set up high-volume manufacturing, and then you need another three years to do durability testing. So even if any of these post-Li and non-Li-ion battery technologies see some breakthrough today, it would be at least six years before they achieve any sort of high-volume production.
References:
Batteries Technology Development Report, technical report by the Joint Research Centre (JRC), the European Commission’s science and knowledge service.
Lithium ion battery value chain and related opportunities for Europe, Science for Policy report by the Joint Research Centre (JRC), the European Commission’s science and knowledge service.
Komentáře